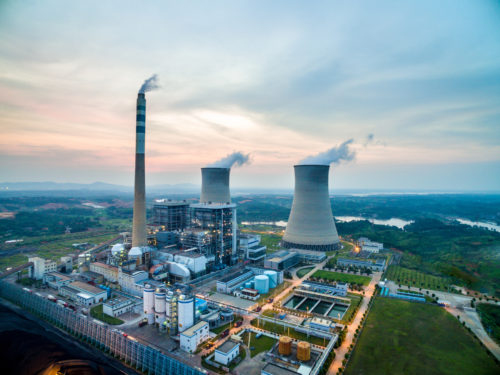
Does “Fuel On Hand” Make Coal and Nuclear Power Plants More Valuable?
On April 14, Energy Secretary Rick Perry sent a memo ordering a 60-day departmental study of whether federal policies favoring an unnamed competitor—evidently renewable electricity like solar and windpower—are constraining supposedly vital “baseload” plants (impliedly coal and nuclear), to the assumed detriment of grid reliability and resilience. Existing studies by grid operators (like PJM, MISO, WECC, and CAISO), their coordinating body, trade associations, numerous academics, the heavyweight Energy Transition Commission, the Department of Energy, and its National Laboratories like NREL have already shown the contrary, though their expert authors are reportedly not being engaged in this new study.
Then-Governor Perry’s highly successful market- and policy-driven efforts helped make Texas by far No. 1 nationwide in windpower (over 20 GW and 15% of 2016 generation) and No. 6 in solar power. Texans benefitted via over 25,000 jobs, economic vitality, and cheaper electricity (hitting record low wholesale prices in 2016 thanks to gas and wind). Secretary Perry emphasized this story in his confirmation hearing, and on March 17, tweeted his justifiable Texas pride at these accomplishments.
Nonetheless, his memo emphasizes the need to diversify fuels rather than to displace fuels; implicitly blames coal and nuclear retirements on renewables rather than on their main cause, fracked natural gas; and adopts a “baseload”-centric view of the grid that most experts abandoned years ago as no longer useful or necessary.
But one feature is truly novel and merits examination: the claim that power stations with “fuel on hand” contribute to “grid resilience” and hence are worth more than their market prices reflect. This notion is so new that a Google search for “fuel on hand” returns zero entries in this context. Without trying to parse what the Secretary might mean by “resilience”—which my 1981 Pentagon-funded study Brittle Power: Energy Strategy for National Security explained in detail—let’s examine whether “fuel on hand” confers distinctive advantages. First we must ask which kinds of power plants have this attribute.
They’re not wind and solar power (together 6.6% of 2016 U.S. generation) because those use no fuel. Their energy source arrives for free, with accurately forecastable variations but virtually no risk of long interruptions. (That risk isn’t exactly zero because of the small but very consequential risk of a gigantic Krakatoa-class volcanic eruption or a postwar “nuclear winter.” Cheap insurance in an all-renewable grid could come from a mothballed “strategic capacity reserve” of scarcely-ever-run fueled power plants, but if such grave contingencies occurred, energy would be far from humanity’s main problem.)
Traditional hydropower (6.5%) may store water behind a dam, but that’s not a fuel either, nor is geothermal heat (0.4%) flowing in the earth’s crust. Among all renewable power sources, the only fuel-burners make 1.5% of U.S. electricity from municipal, industrial, and biomass (farm and forestry) wastes—typically from fairly steady sources located onsite, like a pulp mill, sawmill, refinery, or livestock facility, or nearby, like a landfill.
Gas-fired power plants
The U.S. made 34% of its 2016 electricity from natural gas, chiefly in efficient and flexible combined-cycle gas turbines—almost the only nonrenewable new electric capacity being built in the United States. But typical gas-fired generators don’t keep fuel on hand at the site, relying instead on just-in-time delivery by gas pipeline from remote production and storage facilities (though a few power plants do have associated gas storage and more have multiple pipelines). In principle, power plants could store giant tanks of natural gas or smaller tanks of liquefied natural or petroleum gas (LNG or LPG respectively), akin to traditional fuel-oil storage for plants with dual-fuel capability. But oil isn’t fully reliable, as illustrated in the 2014 polar vortex when 2–3 GW of Northeastern dual-fuel plants were constrained by oil-supply problems, such as scant inventories, limited trucks, gelled fuel, or frozen fuel lines and injectors. Onsite LPG and LNG stocks would be resisted as an extra carrying cost, and LNG boils off if not used.
Fortunately, big pipelines normally deliver natural gas reliably—except when they don’t. Despite rerouting flexibility, natural gas supply via mostly old pipelines is vulnerable to weather and other disruptions, and is inherently vulnerable to physical or cyberattack. More local disruptions can be significant too, like Gulf of Mexico hurricanes. Freezing weather can make fuels unavailable and power plants inoperable, and because natural gas itself is not bone-dry, it can freeze at the wellhead, in the pipelines, and in between.
The North American Electric Reliability Corporation (NERC), the continent’s grid reliability regulator, found that of the 19.5 GW of generating capacity disabled in the 2014 polar vortex, about 91% failed due to equipment frozen in diverse ways that took three pages to list (pp. 14–16). The frigid weather, down to 35F˚ below normal, disabled 8 of 11 GW of gas-fired generators in New England because contractual limitations blocked or exceptional space-heating demand preempted their expected supplies. As electric loads neared or surpassed all-time records, the cold disabled nearly 30% of the mid-Atlantic PJM power pool’s capacity—one-fourth because gas plants couldn’t get gas at any price, the rest mainly because coal-fired plants’ coal-handling equipment or coal piles froze. The Southeast lost 9.8 GW of supply, often because the plants hadn’t been designed for such extreme cold. In the Midwest’s MISO pool, 31% of capacity went offline, a fifth of it because no gas was available. And this wasn’t the first time: the February 2011 Southwest cold snap knocked out power to 4.4 million customers in three states, and in the ERCOT power pool roughly contiguous with Texas, 210 of 550 electric generating units couldn’t start, couldn’t keep running, or ran at reduced capacity.
Natural gas is flammable and explosive, so serious mishaps can occur. The 2000 New Mexico pipeline explosion exacerbated California’s electricity crisis. The 2010 San Bruno explosion and 2015 Aliso Canyon gas storage disaster revealed serious maintenance deficiencies, and their consequences strained California’s gas and electricity supplies. The gas and electricity industries are also mutually interdependent in both supply and demand, so power-grid disruptions could ultimately compromise gas supplies.
NERC concluded that “similar to other energy-limited resources (e.g., wind, hydro), resource adequacy assessments should also consider the fuel and capacity constraints of gas-fired generation.” NERC’s similar concerns about coal deliverability mean that both gas and coal—whose plants had respectively 55% and 26% of generator forced outages in the 2014 polar vortex—need the same sort of reliability thinking as renewables whose output can vary on a shorter timescale.
Cracking the code
Applying to neither renewable nor gas-fired generators, “fuel on hand” is a convenient new codename for coal-fired and nuclear power plants, which respectively generated 30% and 20% of U.S. electricity in 2016. Their advocates sometimes call them “24/7/365” plants, but there is no such thing, because every kind of electricity generator can and does break down occasionally. The average U.S. coal-fired station suffers such a “forced outage” about 6–10% of the time, and for one or another reason is unavailable for about 15% of its theoretical output. The average nuclear plant has a forced outage ~1–2% of the time, plus ~6–7% scheduled downtime for refueling and planned maintenance; a modern and well-maintained combined-cycle gas-fired plant, about 5% of the time; an older condensing gas-fired plant, more.
Such technical failures are far rarer for wind and solar photovoltaic (PV) power. PVs have few moving parts—just tracker motors and inverter fans—and are easily maintained at ground level and at night, so their forced outage rate is close to zero. Modern wind turbines’ forced outage rate is 2% or less—1.8% for nearly 23,000 performance-guaranteed Vestas turbines in 2016, 1.1% in a Sandia database. So these dominant renewables are 98–99% technically available, but varying wind and sun held the average U.S. 2016 capacity factor to 34.7% for windpower (net of several percentage points’ curtailment) and 27.2% for PVs. For comparison, coal was 52.7% and combined-cycle gas 56.0%; renewables are gradually displacing both, while gas is displacing costlier coal.
Does “fuel on hand,” stored onsite in substantial amounts, make fueled power stations somehow more resilient and valuable than other generators? It’s a good question with more claims than analysis, but historical experience may suggest useful insights.
Coal
Two-fifths of U.S. coal use comes from Wyoming’s Powder River Basin (PRB), nearly all via the 103-mile Joint Line rail corridor—the upper left part of the heavy blue line coming out of Wyoming in the map below. In May 2005, heavy rain and snow destabilized that line; two coal trains derailed on consecutive days. Damage to the triple rail line disrupted and reduced shipments for most of the rest of the year, so coal’s spot price more than doubled in five months. The National Academies concluded, “The rail networks that transport the nation’s coal—like air traffic control and electric transmission networks—have an inherent fragility and instability common to complex networks. Because concerns about sabotage and terrorism were largely ignored until recently, existing networks were created with potential choke points [like some rail bridges over major rivers]…that cause vulnerability…[and] the potential for small-scale issues to become large-scale disruptions.” In 2006, the Department of Energy’s Energy Information Administration said: “Hardly a month goes by that delivery of PRB coal somewhere in the supply chain is not interrupted by a derailment, freezing, flooding, or other natural occurrence.” Climate change is likely to increase heat that buckles rails, floods and storms that undermine tracks, and extreme weather that spikes electric demand. Meanwhile, utilities, having cut coal inventories threefold during 1980–2000 to save cost, keep trying to squeeze out more cost, exacerbating risk.
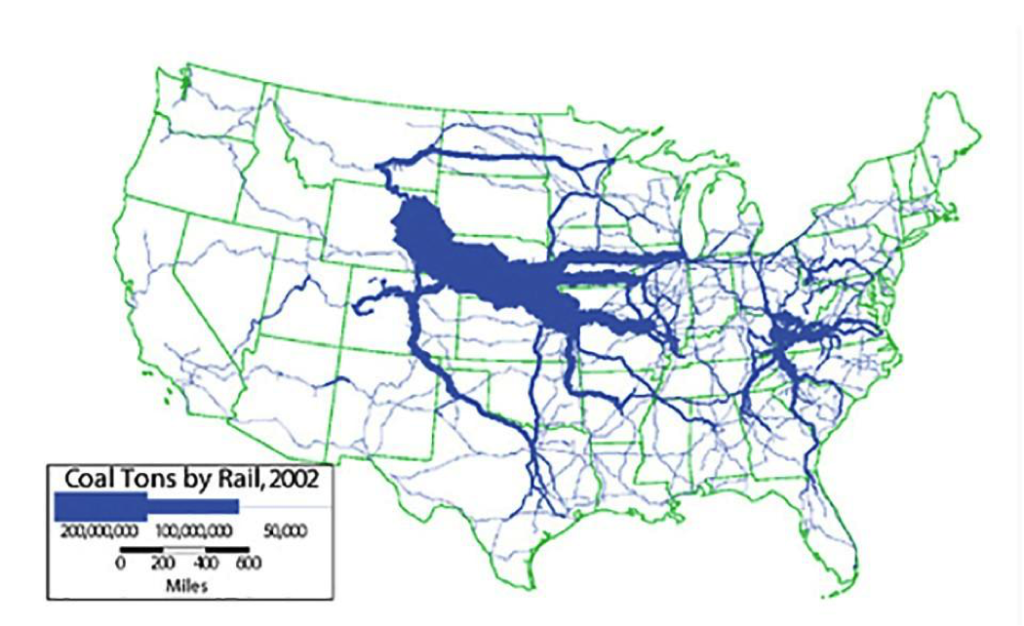
Once coal is delivered, it may not be usable. In February 2011, 50 fossil-fueled power plants in Texas, totaling 7 GW, shut down because of burst pipes, frozen coal piles, and other cold-weather problems they weren’t prepared for. (Some plants were already down for ill-timed routine maintenance.) Many Texas utilities had long promoted electric heat, so they ran short of capacity and called record-length rolling blackouts, which among other things curtailed natural-gas shipments to New Mexico and southern California. (Some commentators blamed windpower, which in fact provided its scheduled 3–4 GW and helped support the overstressed grid, as they did again at the 2016 summer peak.)
In normally cold areas like Pennsylvania and Wisconsin, cold snaps have in various years frozen coal piles, stuck oil barges on frozen rivers, and kept power plants from starting. As weather becomes more volatile, one cannot exclude a repetition of the extraordinary winter of 1917/18, which paralyzed most of the eastern states’ interlocking infrastructure: at one point Baltimore Harbor had three solid feet of ice (p. 202).
Nuclear
Nuclear construction has what drillers call a high “dry-hole risk”—investing money but getting no energy back. Of the 253 U.S. power reactors ordered during 1953–2008, 48% were cancelled or abandoned as uneconomic, 11% were permanently shut down because of intractable reliability or cost issues (or in one case a meltdown), and a further 14% had suffered at least one forced outage of a year or more. When the remaining 68 units (27% of original orders) work well despite their average age of about 37 years, their output is indeed commendably steady and dependable; when they don’t, red ink gushes. Thanks to impressive industry efforts to improve operations, the average U.S. power reactor in 2015 put out 92% of its theoretical full-time full-power output (or, say critics, nearer 84% if deeply troubled plants undergoing major repairs or preparing to shut down were counted too). But nuclear power has five largely or wholly unique reliability issues.
First, routine refueling, usually coordinated with scheduled major maintenance, shuts down the average U.S. nuclear plant for 35 days, typically every ~18–24 months. Once begun, this process must be completed, guessing wrong when scheduling refueling for a period when operator expect low loads can put supply adequacy at risk.
Second, in both Europe and the United States, prolonged heat waves have shut down or derated multiple nuclear plants simultaneously when sources of cooling water got too hot.
Third, a major accident, terrorist attack, or perhaps credible threat at any nuclear plant could cause most or all others in the same country or even in the world to be shut down. All 17 of Tokyo Electric Power Company’s (TEPCO’s) nuclear units were shut down for checks for many months in 2002–04, and some units for several years, after falsified safety data came to light. In 2007, the world’s largest reactor complex, with seven TEPCO units supplying 6–7% of Japan’s power, was completely shut down for 21 months by damage from an earthquake stronger than its supposedly impossible design basis. Seven units at Kashiwazaki-Kariwa produced zero power through 2008, five through 2009. Five units restarted in 2009–11, then all shut down again. Restart of four units is now proposed for 2019–21 but opposed by the governor, who has veto power. The lost output was replaced by recommissioned and hastily finished oil-, gas-, and coal-fired plants; side-effects rippled through Japanese electric bills and world fuel markets. TEPCO ran in the red until 2009. Its extra cost in FY2007 alone was about $5.6 billion. Repairs were reported in April 2009 to have cost $1.6 billion, so the episode’s cost may ultimately total north of $20 billion, straining even the world’s #4 utility.
But meanwhile TEPCO went bankrupt in all but name, crushed by the more than $200 billion cost of the 2011 Fukushima disaster. Political reactions and stricter regulation then shut down all Japan’s nuclear plants. About 34 GW have remained shut for an average of 5.5 years and counting, with only a handful restarted and many if not most likely to be abandoned. Fortunately, of Japan’s total nuclear output lost after Fukushima, 70% (or 64% if not adjusted for GDP growth) were replaced by efficiency, renewables, and other distributed resources in the five years through March 2016. By the time many more reactors could restart, their market may have been snuffed out by more-agile competitors.
Fourth, nuclear outages tend to be prolonged, and problems costly and complex to repair, due to radiation exposure and special materials, procedures, training, and inspection requirements. These factors become more important as plants age. The U.S. has among the world’s oldest nuclear fleets, with 37 units over 40 years old. Also relevant is the challenge of safely storing spent nuclear fuel. With no repository for reliable storage over geologic periods, it’s accumulating at reactor sites, generally in storage pools with serious vulnerabilities. Events at Fukushima reemphasized that at multi-reactor sites (which operators prefer because shared infrastructure and support activities save money), a serious release of radioactivity from one reactor or from damaged fuel storage can prevent operators from keeping other reactors safe.
Fifth, unlike refueling outages scheduled one unit at a time, many reactors can fail simultaneously and without warning in regional blackouts, which necessarily and instantly shut down nuclear plants for safety. But in a often-overlooked attribute unique to nuclear technology, their physics then makes restart slow and delicate: certain neutron-absorbing fission products must decay before surplus neutrons suffice for restart and stable operation. That’s why, after the August 2003 Northeast blackout, the nine affected U.S. reactors could regain less than 3% of their power over the first three days and 41% in the first week, and took two weeks to restore fully (see graph)— hardly a reliable resource. Canada’s restart was even rougher, with Toronto teetering for days on the brink of complete grid failure despite desperate appeals to turn everything off. This characteristic of nuclear plants makes them “anti-peakers”—guaranteed unavailable when they’re most needed.
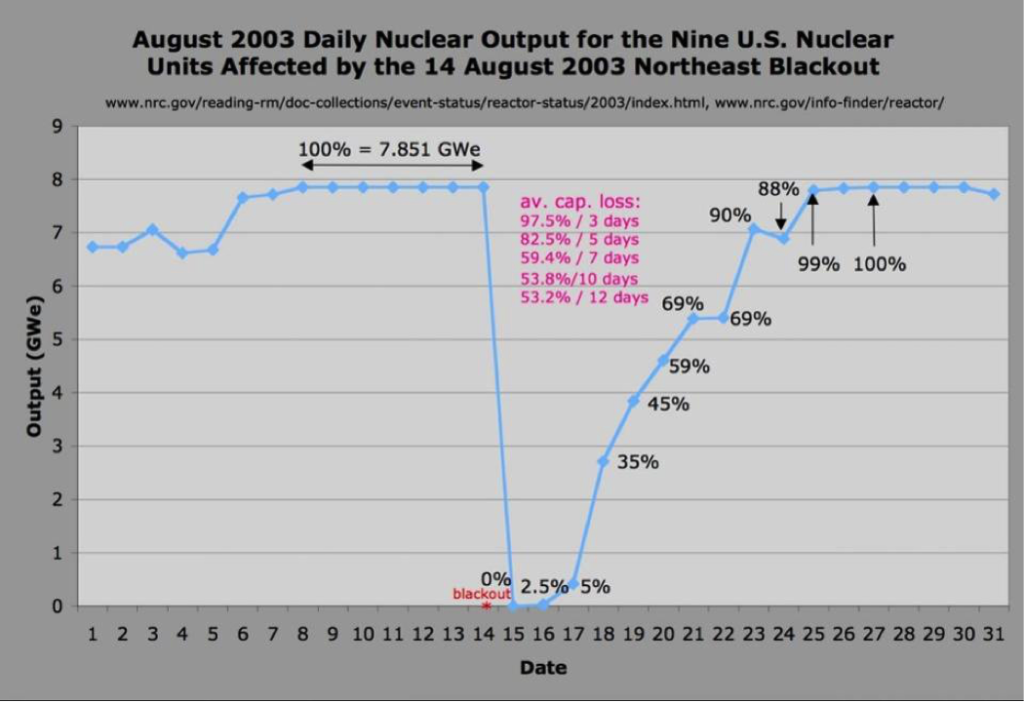
New or even many existing nuclear plants’ inability to avoid carbon emissions cost-effectively makes its troubling reliability attributes seem less worth incurring.
Windpower
Windpower is generally designed for severe weather conditions, and shuts down only if the wind becomes dangerously strong. When the February 2011 cold snap closed dozens of Texas fossil-fueled plants, windpower reliably generated about 3.5 GW during the morning peak. In the 2014 polar vortex, windpower’s consistent output helped save Great Lakes and mid-Atlantic consumers more than $1 billion in two days. In the cold 2013/2014 winter surrounding that polar vortex, wind power provided substantial support to the grid, particularly in Texas, Nebraska, the mid-Atlantic/Midwest, New England, and California. And when New York’s Indian Point 3 nuclear plant failed in December 2015, the state’s wind turbines, augmented by two gas plants, offset that loss.
In South Australia, wind power, blamed by the anti-renewables Prime Minister for causing a statewide blackout on 28 September 2016, has probably made the grid more robust. The past half-century’s worst storm overwhelmed the grid’s redundancy when violent winds, including seven tornados and 80,000 lightning strikes, felled 23 transmission towers and three of the four interconnectors while disabling several gas plants. Wind was producing nearly half the state’s power. When the powerlines failed, gas-fired generation couldn’t have made up the instantaneous loss in windpower, but the blackout could have been averted by another 445 MW of wind—if different software settings to ride through voltage fluctuations had been in place and known to the grid operator, and if sufficient transmission had survived. (Three windfarms kept producing despite the rare pattern of grid faults that stopped six others.) Of course, the failed lines not only cut off all generators including windpower, but would also have cut off a recently closed coal plant had it still been operating, shocking the grid more severely. The state’s blackouts and shortages persisted through the summer despite reliable windpower production using less sensitive settings. A 9 Feb 2017 blackout was caused by gas generators’ failing or not being called upon. More problems still loom due to a gas supply shortage. Producers oversold gas exports, stranding domestic needs including power plants and causing the Prime Minister, to the industry’s dismay, to gain new powers to curtail exports. Such fuel-adequacy issues are no problem with windpower.
Photovoltaics
America’s Armed Services lead the federal government in applying renewable power, especially for effective military operations (pp. 43–49) in forward deployments, where fuel delivery bears a high cost (pp. 33–42) in blood and treasure. Less widely known is that the Services are each installing a billion watts of PV power on or near U.S. military bases, with more to come, to ensure mission continuity even if grid power and fuel logistics fail. PVs also save taxpayer money and stabilize volatile energy prices (which generally make new U.S. gas-fired power plants costlier than renewables).
A major trend in PV deployment, often integrated with other renewable and distributed generators, is to integrate smart load controls with local supplies in “microgrids” that normally interconnect with the surrounding grid but can also isolate (“island”) and stand alone at need. This grid architecture, coupled with efficient use, is the strongest known tool for resilient power supply. In 2009, when a wildfire cut a major power line supplying San Diego, in less than a half-hour the University of California campus’s islandable microgrid, meeting 92% of the campus’s annual electricity needs at $8 million lower annual cost, switched from importing 3 MW to exporting 2 MW of power from its onsite sources, including PVs.
So should we pay more for coal and nuclear power because it has “fuel on hand”?
This evidence about various generators’ reliability suggests six lessons:
- Without exception, all sources of electricity sometimes fail. Their failures differ widely in cause, size, abruptness, predictability, frequency, duration, and importance. Big, lumpy failures are more awkward than small, granular ones.
- Coal- and gas-fired plants’ often-farflung fuel logistics are particularly vulnerable to disruption by weather (worsening with climate change), accident, or malice. Gas and electricity supplies are also interdependent.
- Coal and nuclear power plants, the two kinds that keep “fuel on hand,” are particularly prone to “common-mode failures” that can stop their output over large areas for substantial periods.
- Though nuclear plants require only infrequent delivery of fresh fuel, and accounted for only 3% of the forced outages in the polar vortex, they can suffer relatively infrequent but unusually long outages, can be shut down at a national scale (or more) after certain uncontrollable events, and can be slow and hard to restart after a sudden and widespread blackout, so they too have reliability shortcomings.
- Renewable power has less frequent and briefer technological failures than fueled generation, but windpower and PVs do vary (albeit very predictably) with wind and sun. (Other renewables, delivering half the 2016 global output of all renewables other than big hydropower, are “dispatchable”—you can have them whenever you want.) Especially when integrated into microgrids, renewables are more resilient than generators that need fuel—even windpower and PVs if their forecastable variability is properly managed. That need is analogous to but probably cheaper than managing the intermittence of large thermal plants through reserve margin and spinning reserve. Making largely or wholly renewable power supply highly reliable typically needs little or no bulk electricity storage, but combines proven techniques for forecasting, diversification, integration, demand flexibility, thermal storage, and electricity storage worth buying anyway (such as in parked electric vehicles).
- All these comparisons between generators overlook a very important factor. Whether it comes from a renewable or a nonrenewable power plant, the average electron moves several hundred miles through the transmission and distribution grids before it reaches your meter. But no faraway power plant can serve you if that grid fails. Grid failures, not generator shortfalls, cause roughly 98–99% of U.S. power failures. So if you want the most reliable supply, use a nearby generator, like PVs on your roof, to bypass the grid altogether. If you can’t do that, at least consider a local microgrid to minimize the distance your electricity must travel. If you really want reliable and resilient (Ch. 13) power, no kind of remote central power station is a suitable choice.
Whatever the virtues of fueled central power stations, “fuel on hand” is not one of them. The commendable impulse to diversify power sources does not require substituting one particularly brittle and costly source for another, any more than diversifying a financial portfolio will perform better if you unwisely choose costly and risky investments. To manage both cost and risk, reliability and resilience, a diverse portfolio of efficiency, load flexibility, and renewables is sufficient, smart, and winning in the marketplace—while also advancing free markets, national security, and Creation care.
Both conservatives and progressives who share Secretary Perry’s goals of “reliability, resiliency, affordability, and fuel assurance”—where fuel is needed at all, and not otherwise—will find this a winning formula. Its prudent management of other risks is a free byproduct.
This blog post originally appeared on Forbes.com.